Technology Innovation and Climate Change
- innoveaio
- Oct 1, 2019
- 17 min read
There is no doubt that climate change is one of the greatest challenges of our time. In the last 150 years the concentration of greenhouse gases in the atmosphere has skyrocketed, particularly carbon dioxide, methane and nitrous oxide. We know that these gases accelerate climate change because they trap heat in the atmosphere, thus producing an increase in the earth's average temperature. Professor Rubin explains in this article that environmental problems mostly require joint action to find an effective solution. Voluntary technological policy measures will not be sufficient to stabilize greenhouse gas levels. Sufficiently restrictive regulatory policies are also required to limit emissions and promote technological innovation.
To a large extent, the study of innovation and technological change has been motivated by a desire to understand and shape the forces that underlie economic development and competitiveness in a market economy. Thus, there is a large literature, contributed mainly by social scientists, examining the many facets of innovation and the factors that contribute to it—ranging from the behavior of individuals and organizations, to the role and effectiveness of government policies aimed at spurring innovation in particular sectors of the economy or targeted areas of technology such as computers, aircraft, or agriculture.
The role of technological innovation in addressing societal problems such as air pollution and water pollution is a more recent development. Unlike innovations in industries such as pharmaceuticals or electronics—where the result is new products that consumers desire (such as more effective or lower-cost medicines, cell phones and internet services)—there is little or no “natural” market for most environmental technologies whose function is to reduce or eliminate a pollutant discharge to the environment. Would you voluntarily pay an extra $1,000 to install air pollution emission controls on your automobile if it were up to each consumer to decide? Most individuals would not, recognizing that their action alone would do little to solve the air pollution problem unless all drivers were required to take the same action.
In cases such as this, the role of government policies and regulations becomes critical, since most environmental problems require collective action to effectively address the problems. Similarly, the nature and extent of innovations that lower the cost and/or improve the efficiency of environmental controls depends heavily on the actions of government agencies at all levels.
In this paper we focus on the links between technological innovation and global climate change—which is arguably the most far-reaching and formidable environmental challenge facing the world today. First we present a brief overview of the climate change problem and the innovation needs that motivate this paper. Then we examine in greater detail some of the options available to accelerate the innovations needed to address the climate change challenge. While many of the examples cited in this paper are drawn from experience and studies for the United States, the general concepts and approaches that are discussed are widely applicable to all nations faced with the challenges of climate change mitigation.
The Climate Change Problem
Over the past 150 years, there have been significant increases in the concentration of “greenhouse gases” (GHGs) in the atmosphere, notably carbon dioxide (CO2), methane (CH4) and nitrous oxide (N2O) (see figure 1), as well as a group of industrial GHGs including hydrofluorcarbons (HFCs), perfluorocarbons (PFCs), and sulfur hexafluoride (SF6). Greenhouse gases drive climate change by trapping heat in the atmosphere, which tends to raise the average temperature of the planet. This, in turn, alters the patterns and intensity of precipitation as well as the flows of air and ocean currents around the globe—all of which directly or indirectly influence the climate (defined as the average weather in a region over a period of several decades.)
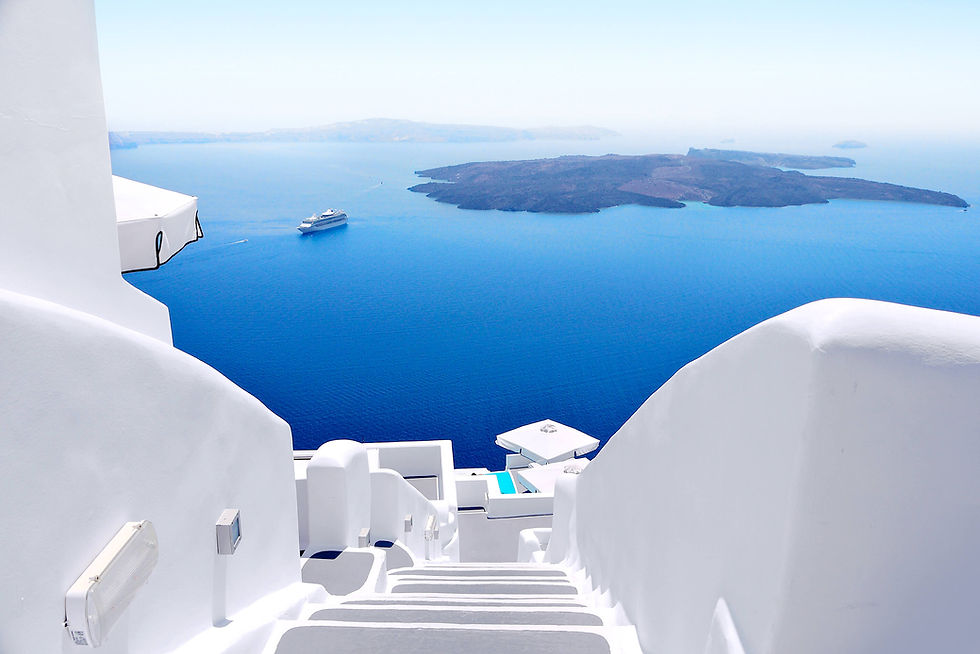
The essence of the climate change problem is that if current trends continue, future global emissions of greenhouse gases will grow significantly in coming decades in response to growth in world population, economic development, and other factors that increase GHG emissions. As a result, the average global temperature is projected to increase by 1.1ºC to 6.4ºC by the end of this century (IPCC, 2007). While there is considerable uncertainty in such projections (as evidenced by figure 3), the potential impacts of global warming could seriously endanger human health, water supplies, agriculture, and human settlements—especially in coastal areas vulnerable to sea level rise and storms (IPCC, 2007b; NRC, 2010b).
In light of these large uncertainties, why not simply wait until there is stronger empirical evidence about the magnitude and impacts of climate change? A fundamental difference between greenhouse gases and “conventional” air pollutants like sulfur dioxide (SO2) and particulate matter is that GHGs, once emitted, remain in the atmosphere for very long periods of time—typically decades to millennia. For example, roughly half the CO2 emitted today will still be in the atmosphere a century from now, still contributing to global warming. Centuries later some of today’s CO2 emissions will still be in the air! In contrast, conventional pollutants like SO2 stay in the atmosphere for relatively short periods of time—typically days or weeks—before they are removed or washed out by various physical and chemical processes. Thus, if we quickly reduced emissions of conventional pollutants their atmospheric concentrations (and associated impacts) also would fall quickly. Not so for GHGs. Because of their long lifetimes, atmospheric concentrations would continue to rise unless emissions were curtailed dramatically. (Think of a bathtub being filled from a large faucet, with only a slow trickle draining from the bottom; the water level would continue to rise unless the faucet were turned down nearly all the way to match the slow drainage.) Thus, if climate change impacts prove to be as serious as projected, reducing GHG emissions in the future would do little to quickly reduce atmospheric concentrations to mitigate those harmful impacts.
What Actions are Needed?
International policy goals for global climate change were established in 1992 under the United Nations Framework Convention on Climate Change (UNFCCC). To date, 192 nations have adopted the UNFCCC goal of “stabilization of greenhouse gas concentrations in the atmosphere at a level that would prevent dangerous anthropogenic interference with the climate system”. Scientific research has sought to better understand and quantify the links between human activities, GHG emissions, the resulting increases in atmospheric concentration, the consequent changes in global temperature, and the impacts of those changes (figure 4). The largest uncertainties are in the links between global temperature increases and resulting impacts. However, based on current science many policymakers worldwide advocate no more than a 2°C rise in long-term global temperature as the climate policy goal needed to prevent dangerous impacts. Achieving that goal would require actions to stabilize atmospheric GHG concentrations at levels only slightly greater than current levels. That, in turn, would require a reduction in annual global GHG emissions of 50% to 80% below 1990 levels by 2050, according to recent studies (IPCC, 2007b).
The technological implications and challenges of meeting such a goal are formidable. This is illustrated in figure 5, which shows the results of recent modeling studies for the United States. These results show there is no unique solution or pathway to achieving large reductions in GHG emissions—different models give different solutions based on different assumptions about the future availability and cost of alternative technologies and other factors. What all models show emphatically, however, is that dramatic changes in the energy system will be required, since this is the dominant contributor to climate change.
Today about 85% of the world’s energy is provided by fossil fuels. Approximately half of that is in the form of oil (used mainly for transportation), followed by roughly equal amounts of coal (used primarily for electricity generation) and natural gas (used for a variety of domestic and industrial heating applications, and increasingly for electric power generation). The CO2 released from the combustion of those fuels—primarily from power plants and automobiles—is the key source of GHG emissions. Achieving a transition to a sustainable low-carbon (ideally zero-carbon) energy system is the major challenge we face to avoid potentially dangerous climate change.
The Need for Technological Change
Technological change on a massive scale will be needed to achieve large reductions in global GHG emissions. The results in figure 5 illustrate the four general strategies available to transform the energy system of a country or region: 1. reduce the demands for energy in all major sectors of the economy (buildings, transportation, and industry), thus reducing the demand for fossil fuels; 2. improve the efficiency of energy utilization so that less fossil fuel is required to meet “end use” energy demands, resulting in lower CO2 emissions; 3. replace high-carbon fossil fuels such as coal and oil with lower-carbon or zero-carbon alternatives such as natural gas, nuclear, and renewable energy sources such as biomass, wind and solar; and, 4. capture and sequester the CO2 emitted by the combustion of fossil fuels to prevent its release to the atmosphere.
As illustrated for the scenario in figure 5 (an 80% reduction below 1990 emissions by 2050), all four approaches are needed to reduce emissions at lowest cost. Reductions in energy demand, which include the effects of improved efficiency, play the most prominent role in all but one of the five models shown. The uncontrolled combustion of coal is eliminated or sharply curtailed in all cases, and the direct use of oil and natural gas also is reduced relative to the year 2000 reference case. In contrast, the use of nuclear power, biomass, and non-biomass renewables (mainly wind) increases significantly in these studies. So too does the use of carbon capture and storage (CCS). This technology could make it possible to capture the CO2 from power plants and other large industrial sources, and then sequester it in deep geologic formations or depleted oil and gas reservoirs. This option has gained substantial worldwide attention in recent years, with efforts now underway to develop and demonstrate the applicability of CCS for climate change mitigation.
The same types of energy system transformations that are illustrated in figure 5 for the United States emerge in other modeling studies at the global level (e.g., IPCC, 2007b; Clark et al., 2009). While energy use is the dominant contributor to GHG emissions, technological change in other sectors will also be needed to deal effectively with climate change. For example, changes in land-use practices, especially deforestation, are needed to reduce or prevent the release of CO2 from natural “sinks” such as forests and soils. Technological change similarly can reduce or avoid emissions of non-CO2 GHGs, such as PFCs in the semiconductor industry or nitrous oxide emissions from the agricultural sector. More broadly, at least some adaptation to climate change will almost certainly be necessary, and such adaptations also will require some degree of technological change (NRC, 2010c).
In short, the development and adoption of new technology is an essential element of any comprehensive response to global climate change. But technological change on the scale required cannot happen overnight. To achieve the substantial reduction in CO2 emissions underlying figure 5, for example, the United States alone would have to retrofit or replace hundreds of electric power plants, tens of millions of vehicles, and hundreds of millions of consumer appliances, building systems (for heating, cooling and lighting), and industrial processes and equipment. Change on this scale will take many decades to achieve.
Many of the technologies needed do not yet exist commercially or are too costly (alternatives to gasoline-powered automobiles is a good example). Some alternatives, such as carbon capture and sequestration technologies for power plants, have yet to gain widespread social and political acceptance. Because the rates of development and adoption of new technologies respond to government policies as well as to market forces such as energy prices, we next look more closely at the processes of technological change and innovation and the factors that influence them.
The Process of Technological Change
As discussed elsewhere (e.g., NRC, 2010a), the general process of technological change can be characterized as involving a number of steps or stages. Different terms are used in the literature to describe these stages, but four commonly used descriptors are:
Invention: Discovery: the creation of new knowledge or new prototypes;Innovation: Creation of a new or improved commercial product or process;Adoption: Initial deployment and use of the new technology;Diffusion: Increasingly widespread adoption and use of the technology.
The first stage—invention—is driven in large part (but not solely) by research and development (R&D), including both basic and applied research. The second stage—innovation—is a term often used colloquially to describe th overall process of technological change. As used here, however, it refers only to the creation of a product or process that is commercially offered; it does not mean the product will be adopted or become widely used. That happens only if the product succeeds in the final two stages—adoption and diffusion, which reflect the commercial success of a technological innovation. Those two stages are the ones that inevitably are most critical to reducing GHG emissions via technological change.
Studies also show that rather than being a simple linear process in which one stage follows another, the four stages of technological change are highly interactive, as depicted in figure 6. Thus, innovation is stimulated not only by R&D, but also by the experience of early adopters, plus added knowledge gained as a technology diffuses more widely into the marketplace. Thus, “learning by doing” (economies in the manufacture of a product) and “learning by using” (economies in the operation of a product) are often (though not always) critical elements that enable the adoption and diffusion of new technologies. Along with sustained R&D (sometimes called “learning by searching”), these stages often help to improve the performance and/or reduce the cost of a new technology—trends that are commonly characterized and modeled as a “learning curve” or “experience curve” (IEA, 2000; McDonald and Schrattenholzer, 2002).
Each stage of the process also requires different types of incentives to promote the overall goal of technological change. An incentive that works well at one stage of the process may be ineffective—or even counterproductive—at another. Large-scale change also must be viewed and considered from a “systems” perspective since the success of any new technology is often dependent upon other technological and non-technological factors. For example, the diffusion of energy-saving technologies that can automatically adjust home appliances like air conditioners and water heaters may depend on the development and dissemination of “smart grid” technology in electrical networks. Similarly, the dissemination of energy-efficient appliances may be inhibited by institutional arrangements, such as landlord-tenant relationships where neither party has an incentive to purchase a more expensive but more energy-efficient appliance. Thus, in addition to technical considerations, the widespread adoption and dissemination of a new technology may require measures to address social and institutional barriers that affect the nature and pace of technological change.
The Importance of Technological Innovation
Any successful strategy to reduce GHG emissions significantly will require actions not only to deploy the low-emission technologies that are available today, but also to foster innovation on new technologies that are needed. Accordingly, there has been growing interest in recent years on ways to foster such innovation, in particular, the role that governments can and should play in that process.
Although research and development is a major element of the innovation process, there is growing recognition that technological innovation is a complex process that commonly involves interactions with other stages of technological change, as depicted in figure 6. Thus, gains from new technologies often are realized only with widespread adoption—a process that usually takes considerable time (often decades) and typically involves a sequence of incremental improvements that enhance performance and reduce costs (Alic et al., 2003).
In the context of this paper, a key question is: what strategies and policies can most effectively foster technological innovations that help reduce GHG emissions? As discussed earlier, GHG emissions depend mainly on the types of energy sources and technologies used to provide the goods and services that society seeks. Thus, technological innovations can help reduce GHG emissions in a variety of ways (NRC, 2010). For example:
New or improved technologies can enable devices such as vehicles, machinery and appliances to use energy more efficiently, thereby reducing their energy use and GHG emissions per unit of useful product or service (such as a vehicle-mile of travel or a lumen of lighting for illumination).New technologies can create or utilize alternative energy carriers and chemicals that emit less GHG per unit of useful product or service (such as renewable energy sources or new low-nitrogen fertilizers).New technologies can create alternative ways of providing goods and services that are less GHG-intensive (such as by using substitute products or materials that have lower GHG emissions, or by facilitating larger system-wide changes such as replacing automotive and air travel with teleconferencing and telecommuting).
Technological innovations can facilitate this full spectrum of possibilities. An even broader set of innovations would include social and institutional systems and designs. For example, innovations in urban planning and development could help reduce future energy demands (and associated GHG emissions) for transportation as well as in residential and commercial buildings. Institutional innovations could provide incentives for electric utility companies and others to invest in measures that reduce the demands for energy, as opposed to policies that favor increased energy sales.
Figure 7 shows one estimate of how technological innovations can reduce the future cost of reducing GHG emissions. In this modeling study, a “business as usual” case—which includes historical rates of technological improvements—is compared to a case with more rapid technological change. The cost of meeting a stringent emission-reduction scenario is reduced dramatically when “advanced technologies” are available. This reduction in the unit cost of abatement translates into large national and global cost savings, especially as emission-reduction requirements grow more stringent over time.
The Critical Role of Government Policy
A major challenge in reducing GHG emissions is that few if any markets exist for many of the more efficient and low-emission technologies that are needed. What electric utility company, for example, would want to spend a large amount of money on carbon capture and storage technology if there is no requirement or incentive to significantly reduce CO2 emissions? How many individuals would willingly buy an advanced electric vehicle that costs much more than a conventional automobile simply to reduce their carbon footprint? Costly actions by firms or individuals to reduce their GHG emissions provide little or no tangible value to that firm or person. Only by government actions that either require or make it financially worthwhile to reduce GHG emissions are sizeable markets created for the products and services that enable such reductions. Government actions to create or enhance markets for GHG emission-reducing technologies are thus a critical element of the technological innovation process.There is no doubt that climate change is one of the greatest challenges of our time. In the last 150 years the concentration of greenhouse gases in the atmosphere has skyrocketed, particularly carbon dioxide, methane and nitrous oxide. We know that these gases accelerate climate change because they trap heat in the atmosphere, thus producing an increase in the earth's average temperature. Professor Rubin explains in this article that environmental problems mostly require joint action to find an effective solution. Voluntary technological policy measures will not be sufficient to stabilize greenhouse gas levels. Sufficiently restrictive regulatory policies are also required to limit emissions and promote technological innovation.
To a large extent, the study of innovation and technological change has been motivated by a desire to understand and shape the forces that underlie economic development and competitiveness in a market economy. Thus, there is a large literature, contributed mainly by social scientists, examining the many facets of innovation and the factors that contribute to it—ranging from the behavior of individuals and organizations, to the role and effectiveness of government policies aimed at spurring innovation in particular sectors of the economy or targeted areas of technology such as computers, aircraft, or agriculture.
The role of technological innovation in addressing societal problems such as air pollution and water pollution is a more recent development. Unlike innovations in industries such as pharmaceuticals or electronics—where the result is new products that consumers desire (such as more effective or lower-cost medicines, cell phones and internet services)—there is little or no “natural” market for most environmental technologies whose function is to reduce or eliminate a pollutant discharge to the environment. Would you voluntarily pay an extra $1,000 to install air pollution emission controls on your automobile if it were up to each consumer to decide? Most individuals would not, recognizing that their action alone would do little to solve the air pollution problem unless all drivers were required to take the same action.
In cases such as this, the role of government policies and regulations becomes critical, since most environmental problems require collective action to effectively address the problems. Similarly, the nature and extent of innovations that lower the cost and/or improve the efficiency of environmental controls depends heavily on the actions of government agencies at all levels.
In this paper we focus on the links between technological innovation and global climate change—which is arguably the most far-reaching and formidable environmental challenge facing the world today. First we present a brief overview of the climate change problem and the innovation needs that motivate this paper. Then we examine in greater detail some of the options available to accelerate the innovations needed to address the climate change challenge. While many of the examples cited in this paper are drawn from experience and studies for the United States, the general concepts and approaches that are discussed are widely applicable to all nations faced with the challenges of climate change mitigation.
The Climate Change Problem
Over the past 150 years, there have been significant increases in the concentration of “greenhouse gases” (GHGs) in the atmosphere, notably carbon dioxide (CO2), methane (CH4) and nitrous oxide (N2O) (see figure 1), as well as a group of industrial GHGs including hydrofluorcarbons (HFCs), perfluorocarbons (PFCs), and sulfur hexafluoride (SF6). Greenhouse gases drive climate change by trapping heat in the atmosphere, which tends to raise the average temperature of the planet. This, in turn, alters the patterns and intensity of precipitation as well as the flows of air and ocean currents around the globe—all of which directly or indirectly influence the climate (defined as the average weather in a region over a period of several decades.) There is no doubt that climate change is one of the greatest challenges of our time. In the last 150 years the concentration of greenhouse gases in the atmosphere has skyrocketed, particularly carbon dioxide, methane and nitrous oxide. We know that these gases accelerate climate change because they trap heat in the atmosphere, thus producing an increase in the earth's average temperature. Professor Rubin explains in this article that environmental problems mostly require joint action to find an effective solution. Voluntary technological policy measures will not be sufficient to stabilize greenhouse gas levels. Sufficiently restrictive regulatory policies are also required to limit emissions and promote technological innovation.
To a large extent, the study of innovation and technological change has been motivated by a desire to understand and shape the forces that underlie economic development and competitiveness in a market economy. Thus, there is a large literature, contributed mainly by social scientists, examining the many facets of innovation and the factors that contribute to it—ranging from the behavior of individuals and organizations, to the role and effectiveness of government policies aimed at spurring innovation in particular sectors of the economy or targeted areas of technology such as computers, aircraft, or agriculture.
The role of technological innovation in addressing societal problems such as air pollution and water pollution is a more recent development. Unlike innovations in industries such as pharmaceuticals or electronics—where the result is new products that consumers desire (such as more effective or lower-cost medicines, cell phones and internet services)—there is little or no “natural” market for most environmental technologies whose function is to reduce or eliminate a pollutant discharge to the environment. Would you voluntarily pay an extra $1,000 to install air pollution emission controls on your automobile if it were up to each consumer to decide? Most individuals would not, recognizing that their action alone would do little to solve the air pollution problem unless all drivers were required to take the same action.
In cases such as this, the role of government policies and regulations becomes critical, since most environmental problems require collective action to effectively address the problems. Similarly, the nature and extent of innovations that lower the cost and/or improve the efficiency of environmental controls depends heavily on the actions of government agencies at all levels.
In this paper we focus on the links between technological innovation and global climate change—which is arguably the most far-reaching and formidable environmental challenge facing the world today. First we present a brief overview of the climate change problem and the innovation needs that motivate this paper. Then we examine in greater detail some of the options available to accelerate the innovations needed to address the climate change challenge. While many of the examples cited in this paper are drawn from experience and studies for the United States, the general concepts and approaches that are discussed are widely applicable to all nations faced with the challenges of climate change mitigation.
The Climate Change Problem
Over the past 150 years, there have been significant increases in the concentration of “greenhouse gases” (GHGs) in the atmosphere, notably carbon dioxide (CO2), methane (CH4) and nitrous oxide (N2O) (see figure 1), as well as a group of industrial GHGs including hydrofluorcarbons (HFCs), perfluorocarbons (PFCs), and sulfur hexafluoride (SF6). Greenhouse gases drive climate change by trapping heat in the atmosphere, which tends to raise the average temperature of the planet. This, in turn, alters the patterns and intensity of precipitation as well as the flows of air and ocean currents around the globe—all of which directly or indirectly influence the climate (defined as the average weather in a region over a period of several decades.)
Technology-policy options
Technology-policy measures can stimulate innovation and help create markets for GHG-friendly technologies by providing incentives and support for the development and deployment of new technology. Table 2 lists a number of available measures, grouped into three categories. The first is direct government support for R&D to generate new knowledge (including new concepts and technologies). This is the most common form of government support for innovation, and typically involves a variety of public and private organizations (Alic et al., 2003; CATF, 2009).
The second column lists additional policy options that directly or indirectly support the development, deployment and commercialization of new technologies. Such measures have had a major impact on technology development in the past. For example, US government procurement of jet aircraft and computers during their early stages of commercialization following World War II was critical to their subsequent development and widespread deployment in the marketplace (Alic et al., 2003). More recently, government support in the form of investment tax credits and production tax credits (or feed-in tariffs) have fueled the rapid growth in wind-power systems, as illustrated in figure 8. Additional measures such as loan guarantees and support for demonstration projects are currently being used to stimulate investments in “clean coal” technologies such as coal gasification and carbon capture and storage systems.
The third group of technology policy options in table 2 reflects measures to stimulate learning and the diffusion of knowledge. These include support for education and training programs, as well as measures such as the development of codes and standards that facilitate the diffusion of new technologies.
Comments